Graduate Student
- FMA
- The Fabricator
- FABTECH
- Canadian Metalworking
Categories
- Additive Manufacturing
- Aluminum Welding
- Arc Welding
- Assembly and Joining
- Automation and Robotics
- Bending and Forming
- Consumables
- Cutting and Weld Prep
- Electric Vehicles
- En Español
- Finishing
- Hydroforming
- Laser Cutting
- Laser Welding
- Machining
- Manufacturing Software
- Materials Handling
- Metals/Materials
- Oxyfuel Cutting
- Plasma Cutting
- Power Tools
- Punching and Other Holemaking
- Roll Forming
- Safety
- Sawing
- Shearing
- Shop Management
- Testing and Measuring
- Tube and Pipe Fabrication
- Tube and Pipe Production
- Waterjet Cutting
Industry Directory
Webcasts
Podcasts
FAB 40
Advertise
Subscribe
Account Login
Search
Engineering Angle: Draw bead restraining forces in sheet metal drawing operations
Researchers analyze the effects of changing draw bead penetration
- By Ashutosh Mokashi, Dr. Sergey Golovashchenko, Natalia Reinberg, Saeid Nasheralahkami, and Yurdaer Demiralp
- December 10, 2020
- Article
- Bending and Forming
In recent years automotive components increasingly have been produced from aluminum alloys and advanced and ultrahigh-strength steels for their excellent structural performance and reduced weight. However, these materials can have more constrained formability than commonly used mild steels.
When automotive stampers design sheet metal drawing processes, they must have accurate information on the distribution of restraining forces, typically provided by a series of draw beads located along the die cavity perimeter. Draw beads have become the most efficient method to provide restraining forces to draw complex-shape components from sheet material without wrinkles and splits.
The restraining forces created by draw beads are the result of bending/unbending the sheet under some level of stretching coupled with friction. The binder force needed to achieve the necessary restraining force is significantly lower when draw beads are used.
According to experimental data from H. Nine, the clamping force is about 80% to 90% of the restraining force with fixed beads. With a flat binder, when restraining force is created by friction only, the binder force needs to be five to seven times larger depending on coefficient of friction (COF). Without draw beads, a larger press size is required to provide sufficient restraining force.
Researchers at Oakland University’s Center of Advanced Manufacturing and Materials (CAMM) analyzed how restraining force can be adjusted by changing the bead penetration. The experimental work was coupled with numerical simulation to demonstrate the applicability of commercial simulation software to predict the required adjustment of the material flow in stamping dies. The experimentally measured restraining force can help determine the bead penetration required in the die design to achieve the assigned line bead restraining force in the simulation.
Experimental Methodology
The draw bead simulator used in this experimental study was constructed around the idea of a sheet metal draw die: The sheet metal flows in between the male and female portions of the draw bead with some clearance, allowing sheet metal to flow outside the bead area (see Figure 1). For this study, the radii on both male and female beads were 4 mm; widths were 9 mm and 13 mm, respectively. The clearance was adjusted by the equalizer blocks, which included a set of calibrated shims separating the flanges of the tool. The hydraulic cylinder held the portions of the simulator clamped to each other. The draw bead simulator was built on a rigid die shoe system (see Figure 2). Clamping force was 34.2 kN.
The researchers used the following experimental procedure:
- Install the draw bead simulator in a 50-kN tensile frame, with the tool weight supported by a steel table bolted to the lower plate of the tensile frame.
- Install the draw bead inserts into the draw bead simulator with the targeted clearance and bead penetration, achieved with calibrated shims.
- Use a feeler gauge to check the clearance between the inserts.
- Lubricate a strip of aluminum alloy 6111-T4 sheet 0.9 mm thick and 50.8 mm wide, clamp its upper end with the upper grip of the tensile testing machine, and clamp the lower portion between the draw bead inserts with a hydraulic cylinder, leaving sufficient material to be pulled through the beads.
- Pull the specimen through the draw beads at the speed of 200 mm/min. for 130 mm.
Numerical Simulation Methodology
Using the simulation software, the researchers developed a numerical model of sheet material flow through the draw bead. A comparison of numerical and experimental results showed that the simulation model, when calibrated, could be used to direct the tryout process of connecting draw bead geometry with splitting, wrinkling, and springback.
Material deformation in the draw beads was simulated in two steps:
- Simulate the strip deformation while closing the beads.
- Simulate the material flow through the beads.
In the model, after the strip was formed by the draw bead in step 1, the same draw bead moved horizontally to the left in step 2 while the sheet was restricted from one side. The results of in-plane force were used to evaluate the corresponding restraining force on the constrained side of the strip.
Results of Experiment and Numerical Simulation
The experimental study was carried out with an adjustable draw bead insert; the advancement of the male bead and the clearance between the flanges of the male and female portions of the tool could be independently changed. The male bead was fabricated as a separate insert with position adjusted by the first set of shims; the gap between the flanges of the draw bead inserts was adjusted by the second set of shims acting with equalizer blocks.
The results on draw bead restraining force for different male bead penetrations and 0.99-mm clearance between the flanges of the male and female beads (leaving 10% clearance of sheet metal thickness between the sheet and the inserts) showed that the restraining force can be varied by approximately a factor of four. This gives the die designer plenty of flexibility to perform a virtual tryout and achieve the desired distribution of restraining forces around the perimeter of the blank by selecting corresponding bead penetration. The material might not fully conform to the shape of the beads at smaller bead penetrations, and the wrapping angle where friction contributes to the restraining force varies significantly with the depth of bead penetration; therefore, an analysis is required for each bead geometry.
The numerical simulation with the software was targeted to define the COF that correlates the numerical restraining force to its experimental value. Results indicated that 0.14 provides the best correlation for all depths of penetration. Figure 3 shows the experimental and simulation results with different bead penetrations from 1 mm to 10 mm.
Contact pressure varied in different locations of the draw bead surface. The positive and negative peaks shown in Figure 4 indicate whether the pressure is applied to the female bead or the male bead. Pressure distribution depends on bead geometry and therefore is not uniform.
Conclusions
The experimental study and numerical simulations indicated that draw bead restraining force can be varied significantly by adjusting the bead penetration. Results demonstrated that numerical simulation can be successfully used to design the distribution of restraining forces if the COF is selected based on correlation with experimental data.
A simple set of tests performed with the draw bead tool can serve as a transition between the line bead simulation selecting the necessary restraining force and the design of the die.
These experimental and numerical studies illustrate a simple way to define the COF for small-radii areas of a stamping die with significant friction.
ReferenceH. Nine, “Draw bead forces in sheet metal forming,” in proceedings of Mechanics of Sheet Metal Forming international symposium, 1978, pp. 179-209.
About the Authors
Ashutosh Mokashi
Oakland University - Center of Advanced Manufacturing and Materials (CAMM)
115 Library Drive
Rochester, MI 48309
248-370-4051
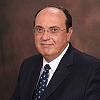
Dr. Sergey Golovashchenko
Professor and Director
Oakland University Center of Advanced Manufacturing and Materials (CAMM)
115 Library Drive
Rochester, MI 48309
248-370-4051
Natalia Reinberg
PhD Student
Oakland University - Center of Advanced Manufacturing and Materials (CAMM)
115 Library Drive
Rochester, MI 48309
248-370-4051
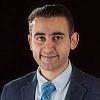
Saeid Nasheralahkami
PhD Candidate
Oakland University Center of Advanced Manufacturing and Materials (CAMM)
115 Library Drive
Rochester, MI 48309
248-370-4051
Yurdaer Demiralp
Graduate Student
Oakland University - Center of Advanced Manufacturing and Materials (CAMM)
115 Library Drive
Rochester, MI 48309
248-370-4051
subscribe now
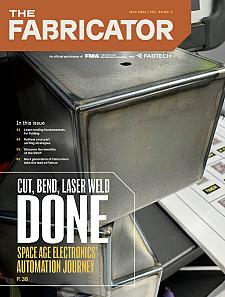
The Fabricator is North America's leading magazine for the metal forming and fabricating industry. The magazine delivers the news, technical articles, and case histories that enable fabricators to do their jobs more efficiently. The Fabricator has served the industry since 1970.
start your free subscription- Stay connected from anywhere
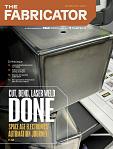
Easily access valuable industry resources now with full access to the digital edition of The Fabricator.
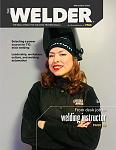
Easily access valuable industry resources now with full access to the digital edition of The Welder.
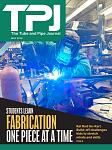
Easily access valuable industry resources now with full access to the digital edition of The Tube and Pipe Journal.
- Podcasting
- Podcast:
- The Fabricator Podcast
- Published:
- 04/16/2024
- Running Time:
- 63:29
In this episode of The Fabricator Podcast, Caleb Chamberlain, co-founder and CEO of OSH Cut, discusses his company’s...
- Industry Events
16th Annual Safety Conference
- April 30 - May 1, 2024
- Elgin,
Pipe and Tube Conference
- May 21 - 22, 2024
- Omaha, NE
World-Class Roll Forming Workshop
- June 5 - 6, 2024
- Louisville, KY
Advanced Laser Application Workshop
- June 25 - 27, 2024
- Novi, MI